Hyperpolarization using parahydrogen
The hyperpolarization of nuclear spins like 13C is a promising approach to access the hidden orders of magnitude of signal in MR imaging and spectroscopy, holding great potential to improve medical diagnostics (1,2). Parahydrogen‑based methods employ the inherent spin order of parahydrogen (pH2) and critically rely on its purity (3-7). For maximum yield, an enrichment of as close to 100 % is demanded prior to any hyperpolarization experiment. pH2 is the spin-singlet isomer of dihydrogen (Fig. 1), which has four spin states in total (one para, three ortho (oH2)). Following the Boltzmann distribution, these four states are approx. equally populated at room temperature (rtH2), i.e. rtH2 = 25 % pH2 and 75 % oH2. At lower temperatures, the equilibrium is shifted towards the para-isomer, reaching a pH2 enrichment fraction (fpH2) ~ 100 % close to the boiling point of H2 (20 K). While the mechanism of interconversion between both fractions is still being discussed (8,9), the life time of para-enriched hydrogen generally exceeds hours or days, dependent on its interactions with the storage container.

Figure 1: Spin isomers of dihydrogen: three-spin ortho- or triplet states (S = 1, oH2) and one para- or singlet state (S = 0, pH2). Equally populated at room temperature, parahydrogen enrichment of the order of unity is achieved at low temperature, and pH2 gas can be produced in large quantities. As an S = 0 spin particle, pH2 does not give a NMR signal; all H2 MR signal stems from oH2.
The spin properties of H2 have been the subject of discussion since the early days of quantum mechanics (10-12) and several methods were suggested for the production of parahydrogen for various applications (e.g. (13-15)). All rely on contact with a catalyst at low temperatures (e.g. liquid N2 at 77 K, fpH2(77 K) ~ 50 %). Recently, some experimental setups have been described aiming at the production of pH2 for hyperpolarization (16,17). Generally, pH2 production is not trivial as H2 poses a considerable safety risk, being highly explosive when mixed with air at 4 % ‑ 76 %, and aggressive to some materials (causes hydrogen embrittlement). Furthermore, the previously described designs and procedures are (a) relatively low in production rate (less 1 standard liter per minute, ls/m, SI units), (b) low in pressure (less 10 bar), (c) low in enrichment (50 %) or (d) can’t be translated easily to a hospital (e.g. access to an NMR spectrometer, or continuous scan times exceeding 50 hours). These may be the reasons why the implementation and operation of a production unit in a clinical setting, to our knowledge, has not been attempted yet.
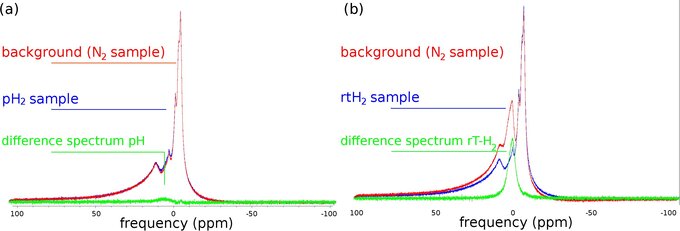
Figure 2: Quantification of pH2 enrichment fraction: 1H - MR spectra of glass vials filled with N2 (red, background), pH2 (blue, left) and room temperature H2 (blue, right). By subtraction of the background (red), the signal of both samples is isolated (green, SpH2, SrtH2), allowing the quantification of the pH2 enrichment: fpH2 = 1 0.75 • SpH2 / SrtH2 = 98 % (pulse-acquisition sequence, NA = 256, TR = 200 ms, B0 = 7 T). The background signal originates from the materials of the transmit-receive coil.
In the scope of hyperpolarization’s application in life sciences, however, this is a prerequisite: future applications are likely to be found in the vicinity of medical research, e.g. in hospitals, where the production of pH2 is severely restricted. It is the aim of this contribution to lower this hurdle, which represents but one on the way to routine clinical use of pH2 hyperpolarization methods.
We developed a high-throughput, high-pressure continuous-flow parahydrogen converter exceeding the specification of its predecessors (Fig. 2). Furthermore, we present its safety concept, quality assurance and routine operation protocols with the goal to supply pH2 safely for subsequent biomedical hyperpolarization research in medical institutions.
The results of this project were published in:
Hövener J-B, Bär S, Leupold J, Jenne K, Leibritz D, Hennig J, Duckett S, v. Elverfeldt D: “A continuous-flow, high-throughput and –pressure pH2 converter for hyperpolarization in a clinical setting”, NMR in Biomedicine, 2012, in press, DOI: 10.1002/nbm.2827
References
1. Bhattacharya P, Chekmenev EY, Perman WH, Harris KC, Lin AP, Norton VA, Tan CT, Ross BD, Weitekamp DP. Towards hyperpolarized 13C-succinate imaging of brain cancer. Journal of magnetic resonance 2007;186:108-113. <bf>2. Roth M, Koch A, Kindervater P, Bargon J, Spiess HW, Munnemann K. (13)C hyperpolarization of a barbituric acid derivative via parahydrogen induced polarization. J Magn Reson 2010;204(1):50-55. <bf>3. Bowers CR, Weitekamp DP. Para-Hydrogen and Synthesis Allow Dramatically Enhanced Nuclear Alignment. Journal of the American Chemical Society 1987;109(18):5541-5542. <bf>4. Chekmenev EY, Hövener J-B, Norton VA, Harris KC, Batchelder LS, Bhattacharya P, Ross BD, Weitekamp DP. PASADENA Hyperpolarization of Succinic Acid for MRI and NMR Spectroscopy. Journal of the American Chemical Society 2008;130:4212-4213. <bf>5. Golman K, Axelsson O, Johannesson H, Mansson S, Olofsson C, Petersson JS. Parahydrogen-induced polarization in imaging: Subsecond C-13 angiography. Magnetic Resonance in Medicine 2001;46(1):1-5. <bf>6. Bowers CR, Weitekamp DP. transformation of symmetrization order to nuclear-spin magnetization by chemical-reaction and nuclear-magnetic-resonance. Physical Review Letters 1986;57(21):2645-2648. <bf>7. Duckett SB, Newell CL, Eisenberg R. MORE THAN INEPT - PARAHYDROGEN AND INEPT+ GIVE UNPRECEDENTED RESONANCE ENHANCEMENT TO C-13 BY DIRECT H-1 POLARIZATION TRANSFER. Journal of the American Chemical Society 1993;115(3):1156-1157. <bf>8. Buntkowsky G, Walaszek B, Adamczyk A, Xu Y, Limbach HH, Chaudret B. Mechanism of nuclear spin initiated para-H-2 to ortho-H-2 conversion. Physical Chemistry Chemical Physics 2006;8(16):1929-1935. <bf>9. Wigner E. Concerning the paramagnetic conversion of para-ortho hydrogen. III. Zeitschrift Fur Physikalische Chemie-Abteilung B-Chemie Der Elementarprozesse Aufbau Der Materie 1933;23(1/2):28-32. <bf>10. Heisenberg W. The quantum theory of line structure and the anomalous Zeeman effect. Zeitschrift Fur Physik 1921;8:273-297. <bf>11. Born M, Heisenberg W. The quantum theory of molecules. Annalen Der Physik 1924;74(9):1-31. <bf>12. Bonhoeffer KF, Harteck P. Characteristics of parahydrogen. Zeitschrift Fur Elektrochemie Und Angewandte Physikalische Chemie 1929;35:621-623. <bf>13. Tam S, Fajardo ME. Ortho/para hydrogen converter for rapid deposition matrix isolation spectroscopy. Review of Scientific Instruments 1999;70(4):1926-1932. <bf>14. Hövener J-B, Chekmenev EY, Norton VA, Weitekamp R, Harris KC, Perman WH, Robertson L, Weitekamp DP, Ross BD, Bhattacharya P. PASADENA hyperpolarization of 13C biomolecules: Equipment design and installation. Magnetic Resonance Materials in Biology, Physics, and Medicine 2009;22(2):111-121. <bf>15. Tom B, Bhasker S, Miyamoto Y, Momose T, McCall B. Producing and quantifying enriched para-H2. Review of Scientific Instruments 2009;80(016108):1-3. <bf>16. Feng B, Coffey A, Colon R, Chekmenev EY, Waddell K. A pulsed injection parahydrogen generator and techniques for quantifying enrichment. Journal of magnetic resonance 2011;in press. <bf>17. Gamliel A, Allouche-Arnon H, Nalbandian R, Barzilay C, Gomori J, Katz-Bull R. An Apparatus for Production of Isotopically and Spin-Enriched Hydrogen for Induced Polarization Studies Applied Magnetic Resonance 2010;39(4):329-345.
Dr. Andreas B. Schmidt
Head of Hyperpolarization
Tel.: +49 761 270-93880
E-Mail: andreas.schmidt@uniklinik-freiburg.de
University Medical Center Freiburg
Dept. of Radiology · Medical Physics
Killianstr. 5a
79106 Freiburg